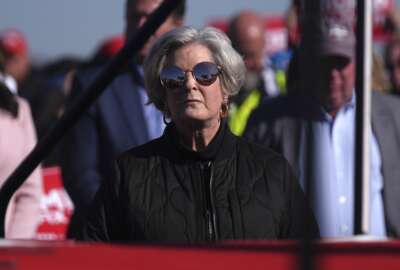
A form of acquired brain injury that occurs when trauma causes damage to the brain, TBI has become increasingly apparent in military personnel.
This content is provided by ICON.
Each year, approximately 69 million individuals worldwide suffer a traumatic brain injury (TBI),1 which is emerging as a major cause of disability and death, as well as a huge burden on healthcare systems and economies. A form of acquired brain injury that occurs when trauma causes damage to the brain, TBI has become increasingly apparent in military personnel. Since 2000, the Defense and Veterans Brain Injury Center reported nearly 350,000 TBI diagnoses in the U.S. military.2
Within this group, TBI is most notably connected to the wars in Iraq and Afghanistan — which accounted for approximately 20 to 25 percent of combat casualties3 — due to more frequent use of improvised explosive devices, suicide bombers, land mines, mortar rounds and rocket-propelled grenades than in prior military conflicts. However, military personnel can acquire TBI in a variety of ways, including recreational activities, falls, motor-vehicle accidents, exposure to explosive blasts and physical training practices such as combatives, boxing competitions and ropes courses.4 In fact, greater than 80 percent of TBI may happen in training, as opposed to active duty.5
Further, for those currently in the military who, prior to enlisting, participated in contact sports — such as football — there is a greater likelihood of overlooked or undiagnosed brain injury that could worsened over time. Factoring in this additional complication, we need to gain a better understanding of military-related brain injury to determine which aspects of service are most strongly correlated with TBI and other negative outcomes. This is important because, similar to civilian populations, studies have reported that mild TBI (mTBI) constitutes the largest number of TBI in military personnel.6
As these populations age, mounting evidence is emerging that TBI is a risk factor for later neurological and neurodegenerative disease — such as Alzheimer’s, Parkinson’s and amyotrophic lateral sclerosis — with studies even indicating there is an increased risk of dementia after a single moderate-to-severe TBI.7 The long-term effects of TBI are also concerning as moderate-to-severe injuries are shown to have had an enhanced risk of late mortality.
Under the circumstances, a clearer understanding of the challenges associated with TBI, how it manifests, and the use of wearables and sensors in capturing physiological data can lead to better designed military-related TBI clinical trials. This, in turn, can result in novel strategies for preventing injury, helping injured personnel return to active duty and maintaining quality of life.
Challenges in TBI diagnosis
Trauma affects the brain in complex ways, resulting in a variety of symptoms and outcomes.
Despite a clear need for preventative measures and novel diagnostic testing to identify mTBI early, no easy-to-use and objective way of measuring mTBI exists. Because of this, medical professionals regularly overlook early-stage injury, resulting in ineffective treatment.
Current methods for diagnosing military mTBI often rely on using subjective tools and retrospective self-reports, which can overlap with symptoms of posttraumatic stress disorder (PTSD) and depression. What’s more, multiple injuries can create a variety of relevant neurological problems, from cognitive to motor diseases. As such, the variety in symptoms and how patients experience the effects of TBI can cause further difficulties in diagnosis. Consequently, no single test can accurately confirm a TBI diagnosis.
Additionally, CT scans — commonly used to assess severity after an injury has occurred and considered the gold standard for structural brain injuries — are not sensitive to the full spectrum of TBI pathology, including functional brain injuries, such as concussion. Therefore, patients with mTBI often have normal CT scan results.
More importantly, no objective tool exists to guide primary care clinicians in their clinical decision to send a patient to specialised care or to an imaging center, which could have grave consequences on a patient’s future brain health. For these reasons, additional research on how to better prevent and diagnose TBI is required, which could lead to new solutions and improved TBI detection tools for identifying mTBI early.
Challenges to long-term treatment, trial enrollment and continuity of care
Some of the same factors creating challenges in the diagnosis of military-related TBI are also present in, and can adversely impact the type of, long-term treatment. Specifically, the set of symptoms that can follow and persist after TBI may be inaccurately associated with PTSD, chronic stress, dementia and chronic traumatic encephalopathy, thereby affecting treatment options. Further, even with the correct course of treatment, the absence of objective biomarkers can make it difficult to monitor treatment.
In addition, when conducting classic randomised controlled trials, heterogeneity in the TBI patient population can cause enrollment challenges. This variability — such as accounting for a helmet used for protection, the location and intensity of the trauma itself, and the initial medical care of each patient — makes it very difficult to define a comparable, yet, not too large, sample.
Another challenge in treating TBI is continuity of care, as deployments happen frequently for both providers and patients within the military. Deployment for providers also means that they may not be able to continue research outside the confines of a specific region. Despite these roadblocks, adopting new strategies can create opportunities for improving military-related TBI clinical trials via novel devices and diagnostics.
Combating challenges in TBI with novel devices and diagnostics
Wearables and sensors can add great value in capturing physiological data that are clinically significant for military personnel living with TBI. Connected digital health technology and electronic patient-reported outcomes enable the remote collection of data outside the clinic. To successfully operationalise digital trials, careful consideration should focus on three main areas: patients, devices and data.
Patients: The combination of military training and outside activities — such as off-duty, sports-related traumas — can put TBI patients at a higher risk for further injury. Moreover, within military populations, there exist varying degrees of exposure, depending on rank, such as what special forces personnel may experience or be exposed to versus an officer.
Therefore, when designing a study, sponsors should involve patients in endpoint development. This includes engaging patient groups not only to help choose endpoints, but also to provide feedback on study questions to ensure the right questions are being asked and are understandable, and to ensure that the measures chosen are relevant and can be validated. Having the right patient population mapped to the right endpoints will ensure better clinical trial success than developing a study and attempting to fit patients into a study.
Devices: When implemented into a clinical trial, innovative, tailored devices and diagnostics can aid in the prevention and diagnosis of mTBI. For example, companies, such as Cerora,8 have developed biosensors based on electroencephalogram (EEG) methodology, which, when used with traditional cognitive tests, may allow researchers and clinicians to make better, more informed decisions about disease stage and outcomes.9,10
Other digital technologies may open new doors for acquiring data from easily accessible devices and can lead to new insights into how TBI may manifest in patients. Smartphones, for example, offer great potential to present visual cognitive function tests in a remote setting. In addition, the Apple ResearchKit provides a platform to develop a variety of patient performance tests and assessments, including a number of cognition tests, such as a paced visual serial addition test, a spatial memory test and a reaction time test.11
Other tests can measure reaction times, memory and problem-solving through the playing of video games and the leveraging of smartphone and tablet technology. Finally, eye tracking has been proposed as an objective biomarker for brain injury and concussion in TBI clinical studies.12
Data: Sponsors can apply artificial intelligence and machine learning to TBI studies to either identify behavioural patterns or treatment strategies. By using analytical tools, such as these, to digest large datasets coming from a registry, one can collect key information about combatants’ activities (e.g, through wearables), treatments and outcomes after TBI.
The increasing miniaturisation and affordability of sensors and circuitry has led to innovation in wearable and microsensor solutions for health monitoring in an effort to collect and assess data related to brain function. In fact, a wide variety of tools exists to assess different components and aspects of brain activities, including those that directly measure brain functioning, such as an EEG, and aspects related to brain function such as sleep patterns, gait, cognition, voice and gaze analysis.13
Another advanced solution that can be used for gathering data is avatar physiotherapy, which allows the service member to regularly perform a predefined set of exercises. At the same time, this type of physiotherapy provides immediate feedback to the patient on how to optimise training, while documenting training effects.14
Search and rescue: Adopting a partner for TBI clinical research
Today, many TBI clinical trials cannot operate as they have in the past. Trial success requires sponsors to focus on the patient first, develop the necessary endpoints, and employ digital health technologies with the greatest potential for better assessing prevention and rehabilitation strategies, especially for military personnel suffering from TBI. Sponsors should consider partnering with a CRO that has extensive experience conducting TBI clinical trials, with a focus on digital technology and patient centricity.
Learn more about ICON’s expertise in military-related TBI clinical trials in our white paper: Traumatic Brain Injury: From identifying biomarkers to improving clinical trial efficiency.
References
1 Dewan, MC, et al. (2018). Estimating the global incidence of traumatic brain injury. Journal of Neurosurgery. DOI: 10.3171/2017.10.JNS17352. https://pdfs.semanticscholar. org/39d8/675e119b3d35156b37d572c9653776cd9564.pdf
2United States Department of Defense Worldwide Numbers for Traumatic Brain Injury. 2016 http://dvbic.dcoe.mil/dod-worldwide-numbers-tb
3 Owens BD, et al. (2008). Combat wounds in operation Iraqi Freedom and operation Enduring Freedom. J Trauma. 64: 295-299
4 McKee AC, Robinson ME. (2014). Military-related traumatic brain injury and neurodegeneration. Alzheimers Dement. 10(3 Suppl):S242–S253. doi:10.1016/j.jalz.2014.04.003
5 Missouri S&T, Phelps Health receive $5.1 million from Army to help military address traumatic brain injury. Missouri University of Science and Technology. (2019) https://www.newswise.com/articles/missouri-st-phelps-health-receive-5-1-million-from-army-to-help-military-address-traumatic-brain-injury
6 Defense and Veterans Brain Injury Center. DoD worldwide numbers for TBI. 2019 http://dvbic.dcoe.mil/dod-worldwide-numbers-tbi
7 Gardner RC, Burke JF, Nettiksimmons J, Kaup A, Barnes DE, Yaffe K. Dementia risk after traumatic brain injury vs nonbrain trauma: the role of age and severity. JAMA Neurol. 2014; 71: 1490-1497
8 Cerora. (2020). https://cerora.com/
9 Simon, A.J., Devilbiss, D.M. (2016). Multivariate models of biosensor data to actively assess sports concussion and mild traumatic brain injury. Neurology 86, 011
10 Simon, A.J. & Devilbiss, D.M. (2015). Sports concussion and mild traumatic brain injury assessment is enhanced with portable, non-invasive biosensor arrays. Med. Sci. Sports Exerc. 47, 11
11 Apple Inc. Apple ResearchKit. Accessed 22 April 2020. www.apple.com/uk/researchkit
12 Samadani, U. Will eye tracking change the way we diagnose and classify concussion and structural brain injury. Concussion 1, 1–3 (2015)
13 Bill Byrom, Marie Mc Carthy, Peter Schueler, et al. (2018). Brain Monitoring Devices in Neuroscience Clinical Research, Clinical Pharmacology & Therapeutics. https://pubmed.ncbi.nlm.nih.gov/29574776/
14 Khan O, Ahmed I, Cottingham J, Rahhal M, Arvanitis TN, et al. (2020). Timing and correction of stepping movements with a virtual reality avatar. PLOS ONE 15(2): e0229641. https://doi.org/10.1371/journal.pone.0229641
Copyright © 2024 Federal News Network. All rights reserved. This website is not intended for users located within the European Economic Area.